Astronomy Object of the Month: 2021, March
< previous Archive next >
Can we observe that the magnetic field heats the surrounding gas?
Magnetic fields can do a lot of things. They are even believed to
govern star formation, by supporting the contraction of gas. Not to mention, that the
galactic evolution often depends on them. They are practically everywhere.
And the gas is also everywhere. Would it be possible, then, that the magnetic fields can heat the gas that surrounds them? To find out, a study is
being performed that combines analyses of both X-ray and radio observations of a sample of quite extraordinary spiral galaxies. One of them is
NGC 5236, better known to sky enthusiasts as M83.
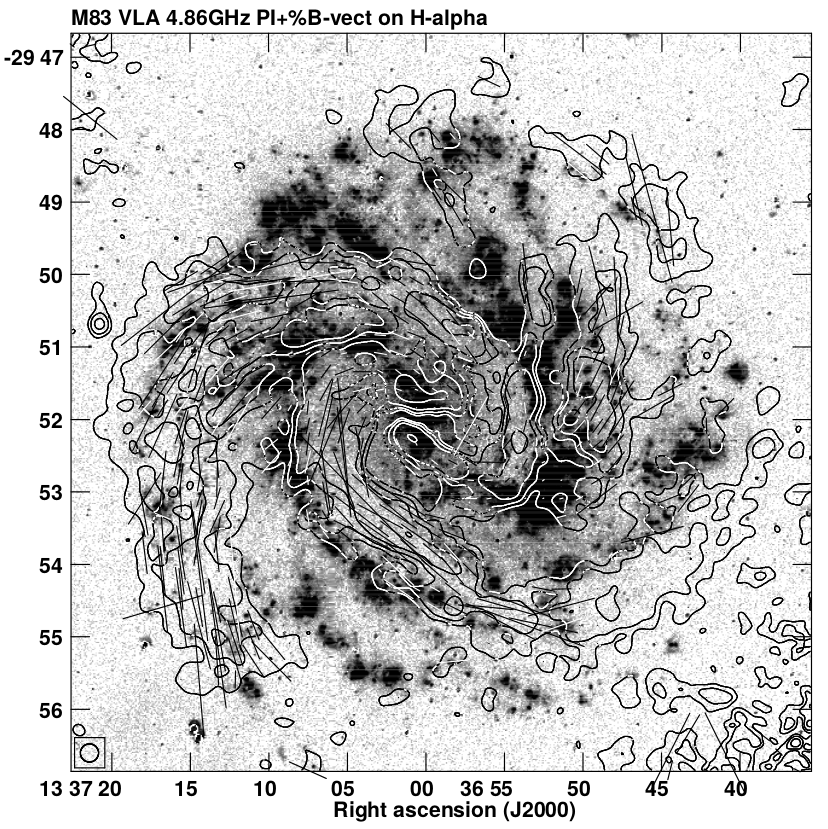
Illustration 1: Halpha; image of M83 with contours of the polarised radio emission and vectors of the magnetic field.
Credit: The Authors.
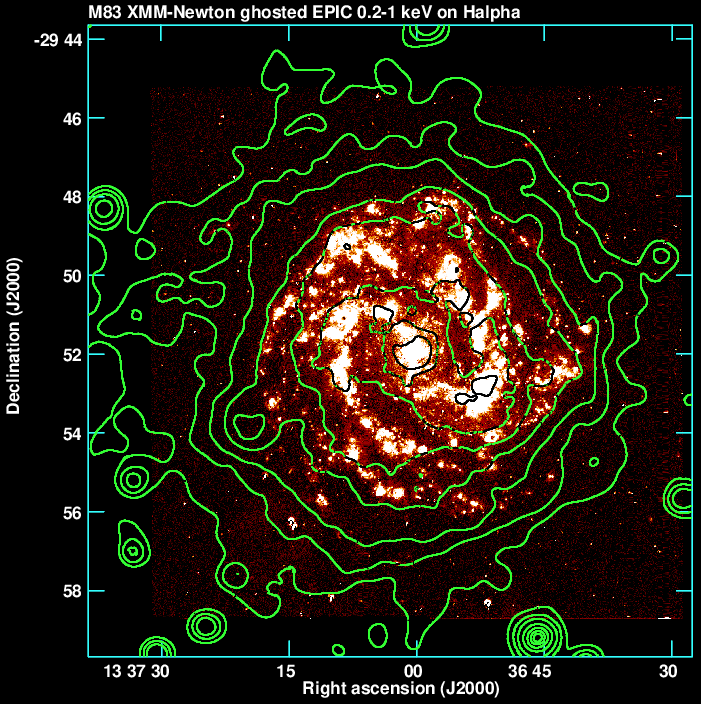
Illustration 2: Halpha; image of M83 with contours of soft X-ray emission. Credit: The Authors.
Many state-of-the-art studies emphasize that the magnetic fields have tremendous influence on the dynamical processes that occur in the interstellar medium (ISM). This influence, however, has mainly a form of the magnetic pressure. Nevertheless, a more direct energy transfer was also considered, having a form of heating of the ISM by magnetic reconnection. It would take place, if the anti-parallel lines of the magnetic field joined and ''dissapeared'', passing their energy to the surrounding particles of gas. The question, of course, is: can we observe this and how could we do it? When the magnetic fields are considered, we need to use radio observations. The heat, properly associated with high energies, leads us to X-ray domain. The main problem with studying this phenomenon is to find the right place to look for such signatures of reconnection heating. Probably the best places might be the so-called magnetic arms, found in several spiral galaxies. These magnetic arms are regions of very well ordered magnetic fields in spiral galaxies that surprisingly are found between the galactic spiral arms consisting of stars, gas, and dust. One could ask, however, why should we look into areas of enhanced magnetic fields if the idea of reconnection heating is based on direct destruction of the magnetic field? This is because reconnection acts mainly on turbulent fields in which we can more easily find "opposite directions" of the field lines. After they are turned into the energy of the surrounding gas, the magnetic field becomes more regular as only the parallel field lines cannot undergo reconnection. As a result, the regions that might host reconnection heating are likely those which show enhanced regular magnetic fields, just like the magnetic arms. What about the heating?
If the gas is expected to be heated, we can try to look for signatures of this heating in X-ray data. The basic assumption is that the X-ray gas (produced mainly by supernova explosions), present in areas devoid of intense star formation, such as the interarm regions (the regions between the spiral galactic arms), should not show temperatures higher (or even comparable) to that found in the spiral arms with vivid star formation activity. If found, such increase in temperature would require an additional source of heating. And the reconnection heating could be such source. The main difficulty in studying these effects is a proper selection of objects. Fortunately, the magnetic arms were found in well-known large spiral face-on galaxies (perhaps this is exactly why they were found). This allowed a detailed X-ray analysis.
One of these galaxies is NGC 5236 or M83, a grand design spiral galaxy. Illustration 1 shows its optical image in the Halpha filter (that nicely shows star-forming regions) with contours of polarised radio emission, hence coming from the ordered magnetic fields, and the vectors representing the orientation of the magnetic field. The length of a vector is proportional to the degree of polarisation, which tells us how strongly is the magnetic field ordered. It is clearly visible that the emission somehow avoids the spiral arms, which means that the magnetic fields are much more random there. Looking at the same optical image but with contours of the X-ray emission from the hot gas (Illustration 2), we instantly see that it is present in the entire disk and beyond. This should not be surprising, as the large and massive M83 is vividly forming stars, which results in large amounts of hot gas produced by supernova explosions is spread across the galaxy and also away from the disk, into the halo.
The spectral analysis of the X-ray emission can provide us with physical parameters of the hot gas, such as its temperature, density, or (thermal) energy. As mentioned above, the main aim of our study is to detect a possible transfer of energy between the magnetic fields and the hot gas. As we expect to find its signatures in the interarm regions, we also need to analyse the areas of the spiral arms to see if any differences can be significant. Because we observe the galaxy from the disk side, the emission that reaches us comes from both the disk and the halo above it. The physical conditions in these areas are different, but we are able to account for that by fitting appropiate theoretical models to the spectra of the X-ray emission. In the case of radio emission from the magnetic fields, we obtain an integrated information from both the disk and the halo. Therefore, a comparison of the X-ray and radio data requires averaging of the derived parameters of the hot gas in the galactic disk and halo.
Having done the necessary data reduction, spectral extraction and model fitting, we obtained energy densities of the magnetic fields and the energies stored in hot gas particles (calculated by dividing the density of the thermal energy by the number density of gas particles) for both spiral arm and interarm regions. For the interarm regions we found slightly higher values of energies per particle, when compared to the spiral arms, accompanied by lower energy densities of the magnetic field. This could be interpreted as a conversion of the energy of the magnetic field into the energy of the surrounding gas, a phenomenon that we expect to be the result of the reconnection of the turbulent magnetic field. Of course, this would also require that the magnetic field becomes more regular (as some part of the turbulent component is removed) and this is exactly what we observe. A good visualisation of the above argumentation is presented in Illustration 3. Its left panel shows the color map of the X-ray intensity with contours of the total radio emission and the vectors of the magnetic field, whose lengths are proportional to the degree of polarisation (and therefore the level of field ordering). We see that the ordered magnetic fields avoid areas of bright X-ray emission -- where the densest turbulent gas is present. The right panel shows the areas used in spectral analysis of the hot gas overlaid on the map of total radio emission. The energies per particle (in parentheses) are higher in interarm regions I2 and I3 than in the spiral arm regions S1 and S3. The highest value found for the spiral arm S2 comes mainly from the highest contribution of the halo emission, which can be explained by vertical magnetic fields that allow the hot disk gas to move more freely up into the halo. It is therefore possible, that the high energy per particle results from reconnection ocurring in these vertical magnetic fields. To verify this hypothesis, reliable and sensitive data at different radio frequency is needed, which will allow to study Rotation Measures in this area and provide direct information about the vertical (thus the line-of-sight) component of the magnetic field.
As shown above, the effects of reconnection heating are likely possible to be traced with present day observations. Nevertheless, currently the analyses must be limited only to angularly large galaxies. Future radio and X-ray telescopes, with higher spatial and spectral resolutions, should allow precise analyses of much larger sample of galaxies, making such studies statistically significant.
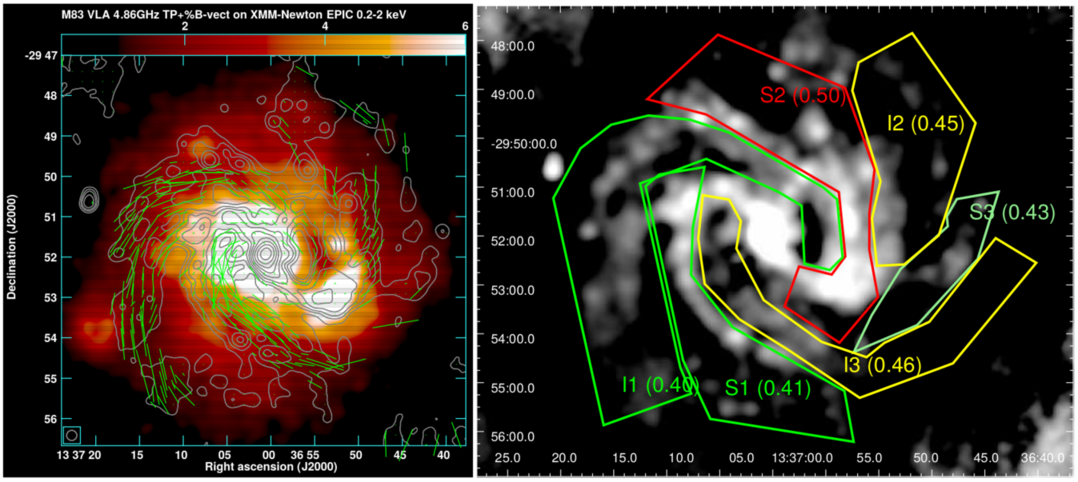
Illustration 3: X-ray intensity map of M83 overlaid with contours of the total radio emission and the vectors with lengths
proportional to the degree of polarisation (left panel) and spectral regions with values of the energy per particle (in parentheses, see the text)
overlaid on the map of the total radio emission. Credit: The Authors.
Original publication: Marek Weżgowiec, Matthias Ehle, Marian Soida, Ralf-Jürgen Dettmar, Rainer Beck, Marek Urbanik, Hot gas heating via magnetic arms in spiral galaxies. The case of M 83, A&A, 2020, 640, 109.
The research was conducted at the Department of Radioastronomy and Space Physics of the Jagiellonian University’s Astronomical Observatory (OA UJ). The work was supported by the Polish National Science Centre through the grant 2017/27/B/ST9/01050.
Marek Weżgowiec Astronomical Observatory Jagiellonian University M.Wezgowiec [at] oa.uj.edu.pl |