Astronomy Object of the Month: April 2024
< previous Archive
Chaos and Einstein-Rosen Gravitational Waves
In 2015, the first detection of gravitational waves was made. Now, nine years later,
gravitational wave astronomy is a rapidly growing field. We have learned to observe
the Universe in a previously unknown way.
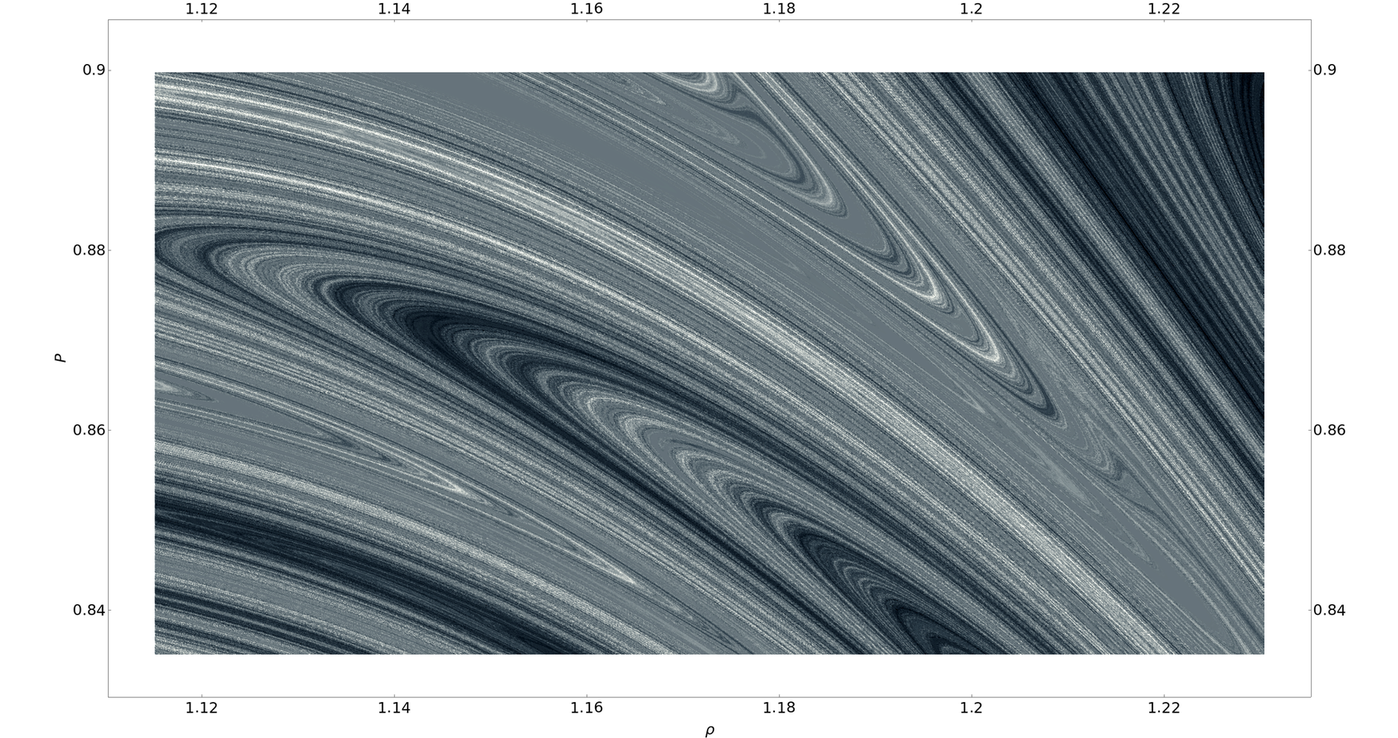
Illustration: The fractal. The parameters on the
axes (P and rho) define the particle's initial state, and the color of the corresponding
dot is associated with its final position obtained after some time. The figure shows that
test particles initially in similar initial states can have entirely different final states. Credit: The Authors.
The success achieved in 2015 did not come out of nowhere. The construction and refinement of the first gravitational wave detector took several decades. The intellectual effort of theorists studying the predictions of Einstein's general relativity had been ongoing since its discovery in 1915. Several generations of researchers over the course of 100 years tried to figure out how to mathematically describe such waves, whether their existence is consistent with the structure of the theory, what properties they have, and how such waves can interact with matter. A key step was the 1937 paper by Albert Einstein and Nathan Rosen. In the original version of this article, the authors erroneously argued that the existence of gravitational waves is inconsistent with the structure of the theory. When their error was pointed out, their article turned into proof of the existence of gravitational waves. The class of mathematical solutions to Einstein's equations found by Einstein and Rosen did not describe waves originating from astrophysical sources. The equations were solved under the simplifying assumption of cylindrical symmetry. Although we know that the universe does not resemble a giant tube, the solutions discovered proved that gravitational waves are admissible in general relativitiy and revealed some of their properties. In their article, Einstein and Rosen mention that the class of solutions they found includes so-called standing waves. This is a special case where the gravitational wave does not effectively move at the speed of light but corresponds to the oscillation of the structure of spacetime like a struck surface of a drum.
Although standing gravitational waves may have appeared in the early universe, there is currently no convincing evidence of their existence beyond the hypothetical possibility arising from the structure of Einstein's equations. Many years ago, experiments were planned in which such waves could be produced in the laboratory and indirectly detected through their interaction with the electromagnetic field. Unfortunately, to this day, these experiments remain beyond our technical capabilities, and the standing waves themselves are the subject of exclusively mathematical research. Although their detection is a matter of the distant future, some of their properties make them truly extraordinary. Stationary vibrations of spacetime in some way resemble particles: localized energy nodes interacting with the environment. If we remember that a gravitational wave is a disturbance of spacetime (time and space), the matter becomes astonishing: time and space, vibrating, effectively behave like an object with mass. In other words, something can be made out of nothing because how else to describe objects made only of time and space? These objects differ from John Wheeler's geons in that they always appear in many copies, like the ridges of small waves on the surface of a drum membrane.
As part of research conducted at the Department of Relativistic Astrophysics and Cosmology, the behavior of test particles in the spacetime of a standing gravitational wave belonging to the Einstein-Rosen class of solutions was examined. Test particles are understood as particles small enough that the spacetime curvature resulting from their energy is negligibly small. Test particles, without disturbing spacetime vibrations, move to their rhythm like grains of sand bouncing on the surface of a drum. The research revealed that the behavior of such particles could be incredibly complex. Deterministic chaos was discovered: although the equations clearly predict the movement of particles, in practice, their final position is extremely sensitive to the initial position. The famous butterfly effect occurs. A detailed analysis of the dynamics of test particles revealed the existence of a complex structure in the solution space known as the heteroclinic network. The butterfly effect for the studied system can be visualized using a fractal. The parameters on the axes (P and rho) define the particle's initial state, and the color of the corresponding dot is associated with its final position obtained after some time. The figure shows that test particles initially in similar initial states can have entirely different final states.
Original publication: The results of the research presented were published in the article: Sebastian J. Szybka, Syed U. Naqvi, “Chaos and Einstein-Rosen gravitational waves”, Phys. Rev. D108, L081501 (2023).
The findings described are part of a study conducted in the Department of Relativistic Astrophysics and Cosmology of the Jagiellonian University Astronomical Observatory in Kraków.
Sebastian Szybka Astronomical Observatory Jagiellonian University S.Szybka [@] uj.edu.pl |